Earth and Climate
elic | Louvain-la-Neuve
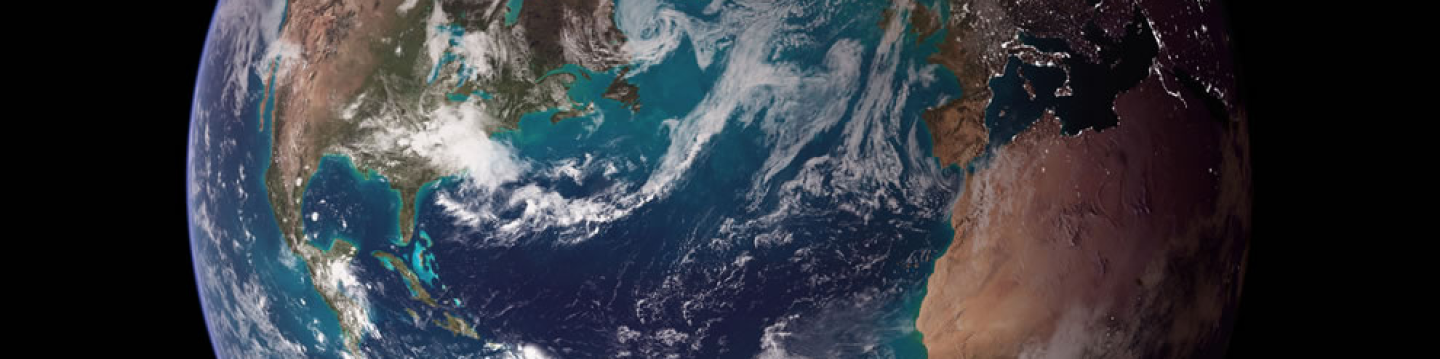
Source NASA, http://pics-about-space.com/
The research conducted in the Earth and Climate Research Center aim at understanding the functioning of the Earth system, with focus on its climate component, and human-environment interactions.